The existence of a vacuum, a space completely empty of matter, had been debated since at least the ancient Greek philosophers, and probably much longer. In 1643 the Italian physicist Evangelista Torricelli showed that for all practical purposes a vacuum was indeed possible.
Philosophical Ideas about a Vacuum
The philosophical debate about the existence of a vacuum, or the void as the Greeks may have called it, has been around for centuries. Is there a void or is no void possible? The debate itself was contentious and much doubt surrounded the idea that a vacuum could actually exist. In ancient Greece the idea began with Democritus, born around 460 BCE, who expanded on and synthesized the work of his teacher Leucippus. Democritus proposed that the world was composed of tiny particles moving around in an infinite void which he called atoms. Thus the space in between these particles was empty space, or what we might today call a vacuum. The most influential Greek thinker, Aristotle, made arguments against these ideas in his book titled Physics. The phrase “horror vacui” (nature abhors a vacuum) is attributed to Aristotle.
Given what we know of the influence of Aristotle over Medieval thinking it is no surprise that the idea of a vacuum fell out of favor during Medieval times. The rejection of a vacuum was restated time and again up until the time of Galileo Galalei.
An Accidental Discovery
Evangelista Torricelli was born in Faenza, Italy in 1608 to a relatively poor family. His father was a textile worker but he had to good sense to realize the extraordinary intellectual talents and abilities of his oldest child. Lacking the resources to provide his son with a proper education he send him to his Jesuit uncle where he was to receive his education at the Jesuit College in Faenza, then later in Rome where he studied science under the Benedictine monk Beneditto Castelli. Castelli was a student of Galileo, a figure who inspired Torricelli’s in his mathematical and scientific career included his experimental verification of a vacuum.
Late in his life, Galileo became preoccupied with the observation that well diggers suction pumps could only raise water about ten meters. In 1640 Galileo, along with his two assistants Torricelli and Giovanni Baliani, conducted a suction pump experiment at a public well. In every instance, no matter what they tried, the water would not rise more than 9.7 meters about the level of the well water. Galileo incorrectly surmised that a force created by a vacuum was preventing the water from rising any higher.
Torricelli discovered the vacuum accidentally when he was conducting experiments that were designed to solve the problem of pumping water out of a deep well. He tried to scale down the problem using mercury instead of water because liquid mercury is much more dense than water and he hoped to be able to observe the same phenomenon at a lower height. He took a tube closed at one end and filled it with mercury. He stuck the open end in a bowl of mercury and slowly raised the closed end, where eventually a gap appeared above the mercury. The gap could not have been air because when he lowered the tube again the gap vanished immediately, quicker than air could have dissipated. The gap above the mercury was the first experimentally verified vacuum.
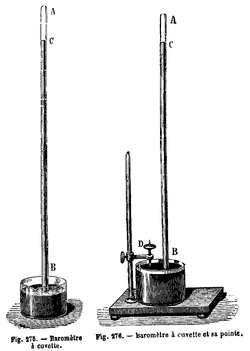
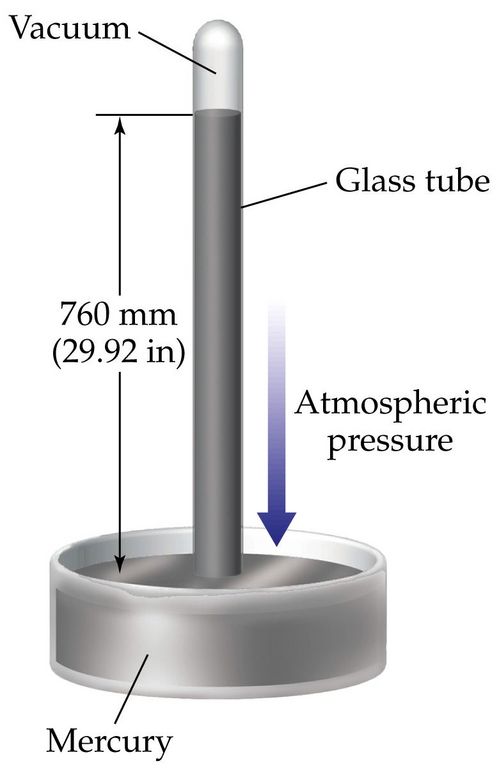
As to the problem of rising water and mercury above a certain level, Torricelli proposed the correct answer. It is that the mercury was rising due to the atmospheres weight (or atmospheric pressure) pressing down on the mercury in the dish. He correctly predicted that height of the mercury column would vary from day to day as the atmospheric pressure also changed. In effect, he invented the apparatus known today as the barometer.
Modern Uses of Vacuum Technology
The discovery of the vacuum was eventually applied to advances in technology and its principle many different industries today. One of the most common uses is in the food industry, where vacuum technology is used in the transport, processing, and packaging of food, and in bottling of beer and soft drinks. It prolongs the shelf life of food and maintains its nutritional content. Vacuum technology is used in the chemical industry to treat and purify reactants and products. Other applications of vacuum technology include usage in heating and cooling systems, light bulbs, steam engines, and cathode ray tubes.
Continue reading more about the exciting history of science!