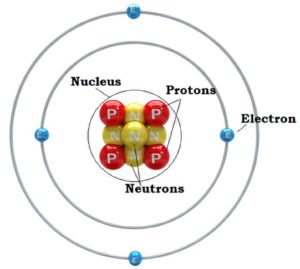
The atomic theory of the atom was proposed by John Dalton in the early 19th century. Dalton claimed that all atoms of the same element are identical, but that atoms of different elements vary in size and in mass. His theory suggested that atoms were indivisible particles, making them the smallest building blocks of matter. However by the middle of the 19th century, a growing body of experiment evidence began to challenge this notion. As the century drew to a close some scientists that speculated that atoms may be composed of additional fundamental units, and by the late 19th century convincing evidence began to emerge from experimental research to support this hypothesis. The discovery of the electron was the first of a series of discovery’s, spanning a few decades, that identified the major subatomic particles of the atom.
J. J. Thompson’s Experiments
This experimental evidence came during the years 1894-1899 when J. J. Thomson conducted research with cathode ray tubes, the same technology that also played a critical role in the discovery of X-rays and on work which led to the discovery of radioactivity. Cathode rays are the currents of electricity observed inside a high vacuum tube. When two electrodes are connected to each end of the tube and voltage is supplied, a beam of particles flows from the negatively charged electrode (the cathode) the positively charged electrode (the anode). In a lecture to the Royal Institution on April 30, 1897, J. J. Thomson suggested that these beams of particles were smaller, more fundamental units of the atom. He termed them ‘corpuscles’ but the name never stuck, and they were eventually given name we are familiar with today: electrons.
J.J. Thomson performed several experiments whose conclusions supported his hypothesis. Firstly, in 1894 Thomson established that cathode rays were not a form of electromagnetic radiation, the assumption at that time, by showing that they much move slower than the speed of light. Soon after he conducted experiments deflecting the rays from negatively charged electric plates to positively charged plates where he was able to show that the beams were streams of negatively charged particles. In another experiment he used magnets to deflect the beams which allowed him to determine their mass-to-charge ration. He approximated their mass at 1/2000th of a hydrogen atom indicating that they must be only a part of an atom. This is an incredibly small mass and is the smallest measured mass of any particle that has mass. Lastly, he showed that these particles are present in different types of atoms.
The revelation that atoms are made of smaller constituent units revolutionized how scientists viewed the atom world and spurred research on nuclear particles. Soon after the atomic nucleus was discovered, and the field of nuclear physics was born. Thomson went on to create one of the first models of the atom, which was called the plum pudding model. He knew that atoms had an overall neutral charge. Therefore, his model depicted the negatively charged electrons floating in a “soup” of positively charged protons. It was a good first attempt at designing a model of the atom but was soon discarded for Ernest Rutherford’s nuclear model of the atom based on the results of his gold foil experiment.
Impact and Legacy
The discovery of the electron had profound effects on both theoretical and applied science.
The discovery of the electron helped to usher in the era of atomic physics and help to give birth to the completely new and foreign field of quantum mechanics. Both of these fields are closely related and describe the behavior of particles at the atomic and subatomic level. Both fields rely on an understanding of atomic structure, of which electrons are a key component.
The discovery of the electron also had a fundamental impact on applied science as it laid the foundation for the development of electronics, a technology that would revolutionize our world. Electrons, being charge carries, are the fundamental working units of electronic components such as capacitors, diodes, resistors, and transistors. They are used in all of the familiar electronic devices such as televisions, smartphones, and computers and have made possible the digital transformation of our civilization. In addition to electronics, electrons are involved in atomic spectroscopy, which is the study of the interaction between light and matter. By studying energy levels and transitions of electrons, atomic physicists can identify elements, determine their properties, and study their behavior in various conditions. Spectroscopy is the method used by astronomers to determine the temperature, chemical composition, luminosity, and other characteristics of distant stars across the universe.
Continue reading more about the exciting history of science!